A novel heat treatment created by MIT alters the microscopic structure of 3D-printed metals, making the materials more durable and resistant to thermal shock. High-performance blades and vanes for jet engines and gas turbines might be 3D printed using the process, opening the door to novel designs with reduced fuel consumption and increased energy efficiency.
Modern gas turbine blades are produced using traditional casting techniques, which include pouring molten metal into intricate molds and allowing it to solidify in a certain direction. As they are made to revolve at high speeds in extremely hot gas, extracting work to generate electricity in power plants and thrust in jet engines, these components are comprised of some of the most heat-resistant metal alloys on Earth.
There is rising interest in producing turbine blades using 3D printing, which, in addition to its financial and environmental advantages, might let manufacturers create more complex, energy-efficient blade shapes more quickly. But a significant obstacle, called creep, still stands in the way of 3D printing turbine blades.
In metallurgy, the term “creep” describes a metal’s propensity to irreversibly change shape when subjected to high temperatures and ongoing mechanical stress. While investigating the printing of turbine blades, researchers discovered that the printing technique results in fine grains with a size range of tens to hundreds of microns—a microstructure that is particularly prone to creep.
According to Zachary Cordero, the Boeing Career Development Professor in Aeronautics and Astronautics at MIT, “in practice, this would mean a gas turbine would have a shorter life or worse fuel efficiency.” These are expensive and unwanted results.
The small grains of the as-printed material are transformed into much bigger “columnar” grains, creating a more durable microstructure that should reduce the material’s potential for creep as the “columns” are aligned with the axis of greatest stress, according to Cordero and his colleagues. The approach, which the researchers describe in today’s issue of Additive Manufacturing, paves the path for the commercial 3D printing of gas turbine blades, they claim.
“In the near future, we envision gas turbine manufacturers will print their blades and vanes at large-scale additive manufacturing plants, then post-process them using our heat treatment,” Cordero says. “3D-printing will enable new cooling architectures that can improve the thermal efficiency of a turbine, so that it produces the same amount of power while burning less fuel and ultimately emits less carbon dioxide.”
Cordero’s co-authors on the study are lead author Dominic Peachey, Christopher Carter, and Andres Garcia-Jimenez at MIT, Anugrahaprada Mukundan and Marie-Agathe Charpagne of the University of Illinois at Urbana-Champaign, and Donovan Leonard of Oak Ridge National Laboratory.
Start of a transition
The novel technique developed by the researchers is a type of directional recrystallization, a heat treatment in which a material is moved at a regulated speed through a hot zone to combine the material’s numerous small grains into larger, more stable crystals.
More than 80 years ago, directional recrystallization was developed, and it has since been used on wrought materials. The MIT researchers modified directed recrystallization for 3D-printed superalloys in their latest study.
The approach was tested using nickel-based superalloys that were 3D printed; these metals are generally cast and utilized in gas turbines. In a series of tests, the scientists positioned 3D-printed samples of rod-shaped superalloys beneath an induction coil in a room-temperature water bath. They drastically heated the rods to temperatures ranging from 1,200 to 1,245 degrees Celsius by carefully drawing each rod out of the water and through the coil at various rates.
They discovered that moving the rods across a temperature range of 1,235 degrees Celsius at a precise pace of 2.5 millimeters per hour caused a sharp thermal gradient that changed the material’s printed, fine-grained microstructure.
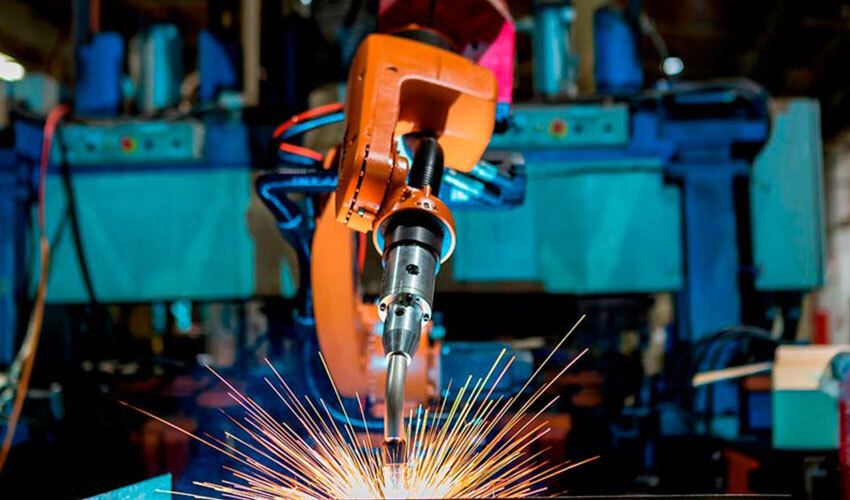
Leave quietly
The printed microscopic grains of the material were replaced with “columnar” grains, or lengthy crystal-like regions, which were substantially larger than the original grains, the researchers discovered after cooling the heat-treated rods.
The structure has undergone a total transformation, according to main author Dominic Peachey. We demonstrate that it is possible to massively increase the grain size to create columnar grains, which should, in theory, result in a significant improvement in the creep qualities.
The scientists additionally demonstrated how they could control the temperature and draw speed of the rod samples to tune the material’s growing grains and produce sections with particular grain size and orientation. The ability to print turbine blades with site-specific microstructures that are resistant to particular operating circumstances, according to Cordero, is made possi
Using 3D-printed shapes that more closely mimic turbine blades, Cordero intends to test the heat treatment. The team is also testing the creep resistance of heat-treated structures and looking into ways to accelerate the draw rate. Then, they think that the heat treatment would make it possible to use 3D printing in a practical way to create industrial-grade turbine blades with more intricate shapes and patterns.
Read More
- The first radiation belt spotted outside of our solar system is being seen by astronomers
- The Paris Air Show Features Flying Taxis; the Olympics are Next
- Networks of nanowires Learn and retain information like a human brain
- Data on populations will be strengthened by new technology and increased processing capacity.